- Oxford Biomedica (UK) Limited, Oxford, United Kingdom
Abstract
Viral vector-based therapies are effective therapeutics for the correction of several disorders, both in mouse models and in humans. Several pre-clinical studies have demonstrated differences in transduction efficiencies and therapeutic effect between male and female mice dosed with AAV-based gene therapy product candidates. Here, we report gender-specific transduction and transgene expression differences in mice dosed systemically with lentiviral vectors (LVV). Male mice systemically dosed with LVV carrying the reporter gene luciferase showed at least a 12-fold higher expression of luciferase and a higher vector copy number (VCN) in their livers compared with female mice. Lastly, PAHEnu2 male mice dosed with a LVV carrying the human phenylalanine hydroxylase (PAH) transgene were observed to have a higher VCN than their female littermates. These findings suggest that sex-based differences initially observed in AAV-mediated therapies also apply to LVV, but the exact mechanism remains to be determined.
Impact statement
There are many reports of lower expression of adeno-associated virus (AAV) transgenes in female compared to male mice. The reason for this difference has not been explained with different theories proposed. This report is the first report that describes the same phenomenon with a different viral vector, i.e. lentiviral vectors, and suggests universality for the phenomenon, not exclusive to AAV, thus excluding a receptor-specific phenomenon explanation. It is hoped that this report will stimulate other groups working with lentiviral vectors to confirm, or refute, this observation from their own data, and potentially other groups working with viral vectors other than lentiviral vectors and AAV. If confirmed, this report will help understand the reasons for this sex-difference phenomenon in murine models and stimulate specific experimental work to explain the phenomenon. Whether this phenomenon is also observed in humans, this is not currently known, but it should also be investigated in humans.
Introduction
Lentiviral vectors (LVV) are an established platform for gene delivery. LVV integrate into the host’s genome providing long-term expression of the therapeutic transgene. They are the preferred platform for the ex vivo transduction of haematopoietic cells or generation of CAR-T cells in clinical applications with good safety and efficacy profiles with several approved products on the market [1]. There are numerous pre-clinical reports of in vivo delivery of LVV targeting the liver for the correction of metabolic disorders [2–8], however none have yet to be translated into the clinic in contrast to AAV based vectors.
Achieving optimal transduction and therapeutic transgene expression following in vivo delivery is essential for demonstrating the efficacy of gene therapy candidates during pre-clinical studies. In general, laboratory mice are the chosen model to do so for gene therapy products. However, there are multiple reports of reduced transduction efficiency, lower expression of the transgene and product efficacy in female mice compared to male mice in liver-directed gene therapy studies with adeno-associated virus (AAV) [9–12].
Several mechanisms for the observed gender differences in transduction and transgene expression in mice have been proposed [10]. It is unlikely that the observed differences are solely due to differential expression of the AAV binding receptor(s) on liver cells, as gender differences have been demonstrated in mice across different AAV serotypes that bind and enter through unrelated receptors [10]. In addition, whether the transduction efficiencies and transgene expression differences in the livers of male vs. female laboratory mice is strain-specific or serotype-specific is not currently known. It has been suggested that transgene expression difference observed in both genders of mice could be due to specific binding of the AAV to liver proteins in an androgen-dependent pathway [10]. Whether this hypothesis applies solely to AAV based vector or to other vector formats remains to be tested.
With AAV based vectors, several routes of delivery have been evaluated for liver-based gene therapies targeting the liver in both pre-clinical models and in the clinic. Intravenous (IV) administration is by far the simplest route of administration that can be translated from pre-clinical models to patients. In addition to IV administration, portal vein (PV) and hepatic artery injections have also been evaluated, as well as direct administration into the liver tissue.
In this study we evaluated in vivo transduction efficiency of hepatocytes and transgene expression of the liver with LVV in both male and female mice. To our knowledge, such a comparison of transduction and transgene expression of vector-encoded transgenes has not been reported with LVV. Here, we report an increase in the transduction efficiencies and transgene expression in the livers of male laboratory mice compared to female mice following delivery of LVV.
Materials and methods
Cell lines
The human embryonic kidney cell line expressing the SV40 large T antigen (HEK293T) was obtained from ATCC (LGC Standards, Teddington, United Kingdom) and cultured in Dulbecco’s modified Eagle’s medium (DMEM, Merck Life Science, Dorset, United Kingdom) supplemented with 10% heat-inactivated fetal bovine serum (FBS; ThermoFisher Scientific, United Kingdom), 2 mM L-glutamine (Merck Life Science) and 1% non-essential amino acids (NEAAs; Merck Life Science). HEK293T cells adapted to culture in suspension phase were maintained in Freestyle™ 293 Expression Medium (FS; ThermoFisher Scientific) with 0.1% cholesterol lipid concentrate 250X (CLC; ThermoFisher Scientific) (FS + 0.1% CLC).
Vector construction
Third generation lentiviral vector carrying Gaussia luciferase cDNA (LVV-GLuc) was generated as follows: the coding sequence for GLuc followed by a T2A and the enhanced green fluorescent protein (eGFP) sequences were synthesized by ThermoFisher Scientific and cloned into a minimal self-inactivating, third generation HIV-1 transfer vector downstream of a mouse transthyretin promoter preceded by a synthetic enhancer [13], also referred to as ETpro. OXB-401 was generated by cloning synthetic cDNA encoding the human codon optimized phenylalanine hydroxylase gene (PAH, ThermoFisher Scientific) into a minimal self-inactivating, third generation HIV transfer vector downstream of the ETpro mentioned above. OXB-Null did not contain any coding sequences downstream of the ETpro.
Vector production
Third generation, VSVG-pseudotyped LVV were produced and titrated in HEK293T cells as described before [14] and resuspended in a formulation buffer of Tromethamine, NaCl, Sucrose and Mannitol (TSSM) [15] which was also used as vehicle control.
Mice
All animal studies were carried out by Charles River (CR) Discovery Services and approved by the local ethics committee. For the initial gene transfer study, 5–8 week-old male and female BTBR mice (BTBR T+ Itpr3/J, n = 5/group, The Jackson Laboratory) of an average weight of 25g, were dosed with 1.5 × 1010 TU/kg [intravenously (IV) via the tail vein] or 7.5 × 109 TU/kg [via the intrahepatic portal vein (PV)] of LVV-GLuc (n = 5/group) or with TSSM buffer (n = 1/group) either via IV or PV routes (Table 1). Dosing was performed according to the average weight of the male or female mice respectively. Due to the maximum volume that could be administered by intrahepatic portal vein injection limited to 100 µL compared to the 200 µL by tail vein administration there is a 2-fold lower total dose administered to the mice via this route. The study designs are summarized in Table 2.
Whole body bioluminescent imaging was performed as described before [9, 16] on days 1, 8, 15, 22, 29, and then once every 2 weeks until day 83 post dosing. Two mice each from Groups 2, 4, 6, and 8 were sacrificed at day 29 to determine VCN. D-luciferin was administered (150 mg/kg) intraperitoneally (IP) and 10 min post substrate injection, dorsal and ventral images were taken, and the scaled images quantified.
For the second study, BTBR-Pahenu2/J mice [17], also referred to as Pahenu2) were obtained from The Jackson Laboratory and dosed IV with TSSM buffer (200 µL), OXB-Null (4 × 1010 TU/kg) or OXB-401 (4 × 1010 TU/kg) and observed for the duration of the study (85 days). All animals were euthanized at the end of the studies and the livers collected and snap-frozen.
Genomic DNA extraction from liver tissues
Liver tissues from median and right lobes were weighed and 22 mg to 26 mg were homogenised in PBS using the TaKaRa® BioMasher standard micro homogenizers. Genomic DNA was extracted using DNeasy Blood and Tissue Kit (Qiagen) following the manufacturer’s instructions.
Quantification of integrated vector copies (VCN)
Duplex qPCR (QuantStudio 7, Thermo ABI) was performed on the liver-extracted DNA to obtain LVV integrated VCN per cell as described before [7] using the transferrin receptor protein 1 (tfrc) as a housekeeping gene. The primer probe set for tfrc was purchased from (ThermoFisher catalogue number 4458366) and primer probe set for HIV Ψ were synthesized by ThermoFisher.
The formula to calculate integrated VCN is as follows:
Statistical analysis
Data analysis and generation of graphs was performed using GraphPad Prism v.9 for Windows (GraphPad, San Diego, CA, United States) with descriptive statistics. Normality tests were used to evaluate the Gaussian distribution of data. Normally distributed data were analyzed using ordinary one-way ANOVA, and data not normally distributed were analyzed using Kruskal-Wallis and Dunn’s multiple-comparisons tests.
Results
Long-term expression of LVV-encoded transgenes in mice treated systemically
An initial in vivo gene transfer study was designed to determine the transduction efficiency of LVV carrying the luciferase transgene (Table 1). Mice were dosed with pre-clinical grade preparations of LVV-GLuc, delivered via the intravenous (IV) or portal vein (PV) routes. In vivo imaging was carried out at regular intervals to determine transgene expression over time. LVV-encoded luciferase activity was observed as soon as day 8 and until the end of the study on day 83 (Figure 1A). Two mice, from Groups 2, 4, 6, and 8, were sacrificed at day 29 to determine VCN with the remaining three mice continuing to day 83. Luciferase expression, measured by whole body luminescence, was observed almost exclusively within the anatomical area which corresponds to the liver and was observed across all vector treated groups, males and females, and via both routes of delivery, IV and PV, from day 8 to the end of the study at day 83 (Figure 1A). In contrast, no luciferase expression was observed in the TSSM-treated groups (Figure 1B).
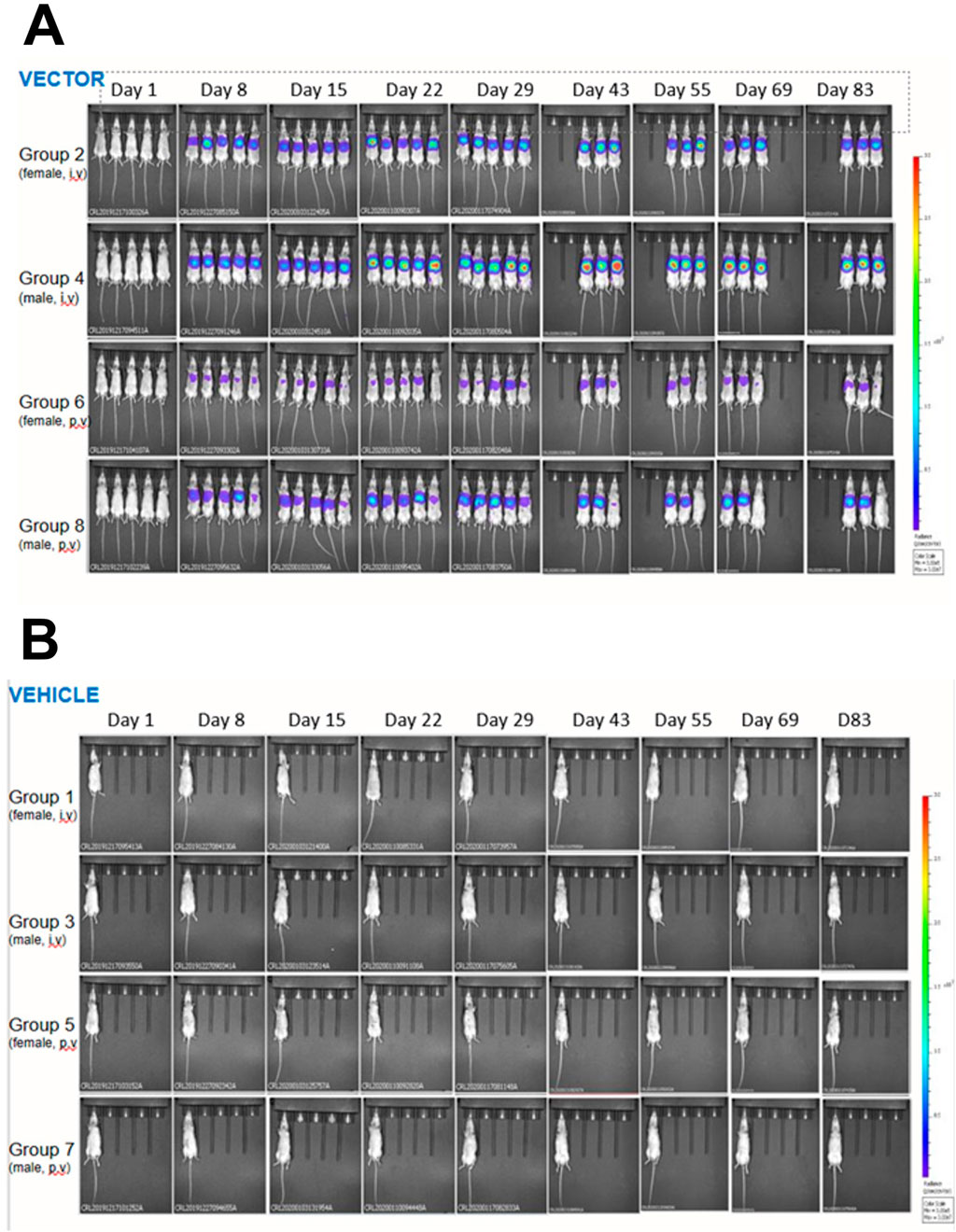
Figure 1. In vivo bioluminescent imaging of the expression of LVV-encoded luciferase in mice dosed with LVV- GLuc (A) or the vehicle TSSM (B) via either the intravenous (IV) or the portal vein (PV) routes. Mice were imaged as described in Materials and Methods.
A quantitative analysis of luciferase expression in vivo showed that the maximum transgene expression was achieved between days 22 and 43 post-dosing and was sustained until the end of the study at day 83 (Figure 2A). Luciferase activity in the TSSM-treated group was considered background activity. In vivo expression of luciferase was higher in the groups dosed IV compared to the groups dosed PV at all time points (Figure 2A). It should be noted that the IV groups had a 2-fold higher dose of vector than the PV groups. A final statistical analysis at the end of the study showed that male mice dosed with LVV-GLuc had higher expression of luciferase than females dosed with the same vector in both IV (12-fold, p = 0.011) and PV (12-fold, p = 0.3867) routes (Figure 2B). In comparing routes of delivery within female and male groups, IV delivery of the vector resulted in higher luciferase expression than PV administration in both females (13-fold, p = 0.0092) and males (13-fold, p = 0.1498).
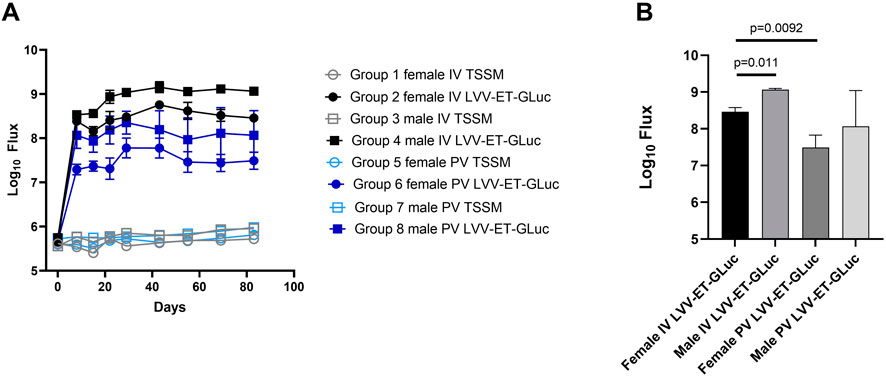
Figure 2. Quantification of luciferase expression of LVV-encoded luciferase in mice dosed with LVV-GLuc. (A) Quantification of in vivo expressed luciferase of all groups over time. (B) Quantification and statistical analysis of the in vivo expressed luciferase of all groups at day 83.
In conclusion, this analysis of the LVV in vivo gene transfer study demonstrated stable transgene expression out to the end of the study at day 83 and that as observed with AAV based vectors there is a higher transgene expression in the male mice compared to the female mice. In addition, the IV route mediated an increase in transgene expression compared to the PV route greater than the two-fold increase in vector dose administered via this route.
Transduction efficiency differences between male and female mice
To determine if the differences in transgene expression observed above were due to differential transduction efficiencies in vivo, integrated vector copy number (VCN) analysis was carried out on the livers of all animals at the end of the study. Two mice from each group were euthanised at day 29 and VCN/cell was measured in the median and right liver lobes. VCN/cell was 4.8-fold higher in males (0.24 VCN/cell) compared to females (0.05 VCN/cell) dosed through the PV route (p = 0.0218) but with mice dosed through the IV route there was no significant difference between the male (0.24 VCN/cell) and female mice (0.21 VCN/cell, p = 0.3935, result not shown).
In the remaining mice taken to the end of the study at day 83, VCN was higher overall in males compared to females for both IV and the PV routes (Figures 3A, B). VCN obtained from male mice in the IV group was 0.25 VCN/cell and not significantly different to VCN obtained from female mice (0.20 VCN/cell, p = 0.4237, Figure 3A) and similar to that observed in the mice taken at day 29. With the mice administered vector via the PV route there is a 5.9-fold increase in VCN/cell in male (0.176 VCN/cell) versus female mice (0.03 VCN/cell, p = 0.014, Figure 3B), which is consistent with the significant difference seen at day 29. In comparing routes of delivery within sexes, male mice dosed IV had 2-fold higher VCN/cell compared with male mice dosed via the PV route (p = 0.0301, Figure 3C). Similarly, female mice dosed IV had 4.9-fold higher VCN/cell compared with female mice dosed via the PV route (p = 0.0008, Figure 3D).
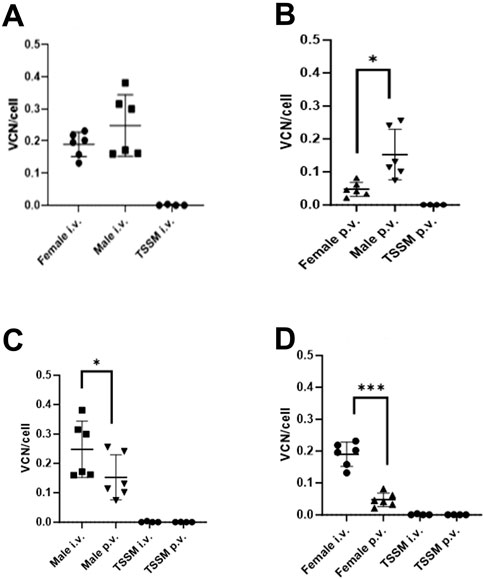
Figure 3. Integrated vector copy number (VCN) in livers of male and female mice dosed via two different routes of administration. Livers were collected at day 83 post dosing. (A) Male vs. female dosed IV. (B) Male vs. female dosed PV; * indicates p = 0.014. (C) Males dosed IV vs. PV; * indicates p = 0.0008. (D) Females dosed IV vs. PV; *** indicates p = 0.0301.
In summary there was a 12 to 13-fold difference in gene expression (from Figure 2 data), a higher VCN in male vs. female mice, and a higher VCN in the IV-dosed vs. PV-dosed groups, therefore we can conclude that the difference in transgene expression between these groups can be partly attributed to differences in transduction efficiencies.
Differences in in vivo transduction efficiencies between male and female mice using a clinically-relevant therapeutic vector and transgene
To determine if the differences in transgene expression observed above were only seen using the reporter gene Gaussia luciferase, an analysis was conducted on a in vivo study using a therapeutic cargo and a mouse model of a human disease. OXB-401 is a third-generation LVV carrying a full-length copy of the human codon-optimized phenylalanine hydroxylase (PAH) open reading frame driven by the liver-specific ET promoter (ETpro). Both male and female Pahenu2 mice (n = 5) were dosed intravenously with OXB-401 or OXB-Null as described in Materials and Methods. VCN analysis was performed on the livers harvested at the end of the study at day 85 days post-dosing. Male mice showed 2 to 3-fold higher but not significant (p > 0.05) VCN/cell than females in both treatment groups (Figure 4).
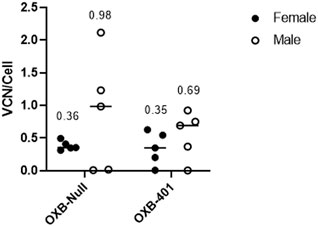
Figure 4. Differential transduction efficiencies in male vs. female Pahenu2 mice dosed IV with either OXB-401 or OXB-Null. Median VCN is shown for each group at day 85 post dosing. No significant differences were observed between the groups (p > 0.05).
When the VCN/cell data in this study were compared with the data from the previous experiment it was observed that male mice had an average of 0.54 VCN/cell and females had an average of 0.25 VCN/cell, however these differences were not statistically significant (p = 0.1792). The spread of the data suggested that the transgene itself (GLuc, PAH or Null) does not have a significant effect on transduction efficiencies (data not shown).
These results are further evidence of the differential LVV transduction efficiencies in laboratory mice.
Discussion
In this report we have shown that the liver expression of a reporter gene administered in vivo using a systemic lentiviral vector was significantly higher in male mice compared to female mice. Vector copy number per cell was also higher in male mice compared to female mice. In addition, an increased trend in transduction between male and female, although not significant, was also observed in a therapeutic model of disease with both the therapeutic vector, OXB-401 and a control vector OXB-Null.
To date there have been no reports comparing the expression of in vivo-delivered LVV between male and female mice. Our initial LVV in vivo gene transfer studies were designed to identify the optimal route of delivery for the transduction of the liver, to assess the safety profile of pre-clinical grade vector batches and to determine duration of the reporter transgene. The current study was designed using both male and female outbred laboratory mice. The luciferase signal was observed within 8 days and peaked between 22 and 43 days post dosing and was stable until the end of the study at day 83 post dosing, indicating long term expression of the transgene following a single administration of the vector. We and others have demonstrated stable transgene expression in other tissues and the liver in pre-clinical models [18]. In the clinic, with a gene therapy for wet-AMD, we have demonstrated stable and long-term transgene expression from a lentiviral vector in the eye for greater than 6 years [19].
In our studies, IV delivery of LVV post 83 days resulted in a 12-13-fold increase in luciferase activity than PV delivery during in vivo imaging in both male and female mice. While there was a two-fold increase in vector dose administered IV compared to the PV route due to constraints in total volumes able to be administered this only partly provides a reason for the increase in luciferase signal. Integrated vector copy number (VCN) analysis, carried out at the end of the study, demonstrated that IV dosing resulted in higher (2 to 5-fold) VCN compared to PV dosing (Figures 3A–D). With AAV based vectors, hepatic artery delivery has been assessed in pre-clinical models [20] and unlike our study they found that intravenous injection of AAV2 resulted in 4-10 fold less hepatic transduction when compared to intra portal injection [21]. For humans, portal vein and hepatic artery injections are more invasive than peripheral intravenous injection and need trained individuals who are experts in interventional radiology or surgical procedures, therefore increasing the complexity of the therapeutic approach compared to an approach based on peripheral injection.
When we compared the transgene expression differences between genders, we observed a higher luciferase expression in mice dosed via the IV route and the PV route post 83 days transduction. The increase was observed as soon as 8 days post dosing in both the IV and PV groups. A similar finding has also been reported with AAV-Luciferase dosed mice [16] This difference in transgene expression has been extensively reported with AAV based vectors in laboratory mice and this is the first report of this observation with LVV.
When VCN/cell analysis was performed at day 29 and day 83 there is a trend of an increase in transduction efficiency in male mice compared to female mice. These increases in VCN/cell may partly account for the increased expression in male versus female mice but other contributing factors may also be involved. To our knowledge this is also the first report of sex-specific differences in LVV transduction efficiencies and transgene expression.
To determine if the differences observed in VCN/cell using the reporter gene luciferase and outbred laboratory mice were specific to this study, a study was conducted using a therapeutic transgene and a mouse model of a human disease. OXB-401 is a third-generation LVV carrying a full-length copy of the human codon-optimized phenylalanine hydroxylase (PAH) gene driven by the liver-specific ET promoter (ETpro). Pahenu2 (BTBR-Pahenu2/J) mice carry a T835C missense mutation, yielding an F263S single amino acid substitution that causes severely diminished PAH catalytic activity in homozygotes and provide a model of severe phenylketonuria. VCN analysis from the livers of Pahenu2 mice harvested 85 days post-dosing demonstrated a higher, but not statistically significant, change in transduction efficiency between male and female mice (Figure 4) with both therapeutic OXB-401 and the OXB-null vectors. Interestingly, two previous publications also reported sex-specific differences in Pahenu2 mice systemically dosed with AAV carrying a human PAH cDNA transgene [11, 22]. These results are further evidence of sex-differential LVV transduction efficiencies in at least two strains of laboratory mice. In our studies we used the liver-specific promoter ETpro, and so it would be important to determine if other promoters result in same or different results.
It has been suggested that androgens are likely responsible for the enhanced AAV transduction in male mice as castration was shown to reduce AAV transgene expression, whereas treatment of female mice with testosterone improved AAV transduction to levels observed in males [10, 23]. Additionally, AAV transduction differences between male and female mice have also been reported in organs other than the liver [24]. Our data indicate that sex-specific differences in AAV transduction efficiencies also apply to VSVG-pseudotyped LVV, but further analysis is required to better understand the mechanism of the gender differences observed in this report, including the effect of LVV envelopes other than VSVG. To date, these gender-specific differences have not been reported in species other than laboratory mice, as most AAV and LVV-related pre-clinical studies only use mice as the model species. When the gender difference expression is also confirmed by other groups, it would be important to assess the relative effects of different LVV envelops. In addition, the effect of age and sexual maturity has not been evaluated in pre-clinical studies. To evaluate gender-specific differences in non-human primates or even humans, the design of relevant studies must be carefully evaluated to obtain statistically significant values. Whether these effects are also relevant in other viral and non-viral vectors, the exact mechanism and whether this also applies to other species, including humans, remains to be investigated. In future studies, it would be of interest to evaluate the efficacy of transduction and transgene expression in in vitro-transduced cells, for example freshly isolated hepatocytes, from male and female subjects. Indeed, if the gender effect is caused by some hormonal factors, the gender difference would not be seen in vitro between female and male cells. Whether there is gender difference on cell lines originally isolated from female or male donors (from either mice of humans) would be a complementary and additional way to tackle this question. On the same line, the inverse in vitro experiments were conducted by Davidoff and colleagues who showed that androgens increased AAV-transgene expression in vitro by upregulating its receptors [10].
With the increase in transduction efficiency observed with AAV based vectors in male mice compared to female mice, numerous initial AAV-related gene therapy studies only used single sex mice thereby increasing the likelihood of observing a therapeutic benefit, with the simplicity and reduce cost of such studies [23, 25–32]. In addition, the effect of sexual maturity has been overlooked so far. Pre-clinical studies using both genders with AAV based vectors are therefore carried out later in the development pathway. Pre-clinical efficacy studies in mice using systemic delivery of LVV are not very common, and the relatively few reports published only use male mice or don’t describe the sex of the animals [2, 3, 6]. Identifying the differences in sex-dependent transduction efficiencies in gene therapy candidates is important because most studies do not factor these differences. A recent report using a therapeutic AAV8 product candidate for MPSIVA where correction was only observed in male mice highlights the importance of using both male and female mice in efficacy studies [12] in that both groups need to be evaluated to inform pre-clinical efficacy of product candidates. Therefore, accounting for sex-specific differences is a critical variable for the development of safe and efficacious AAV and LVV gene therapy candidates.
In summary, we report for the first-time sex-specific differences in LVV transduction efficiencies and transgene expression in pre-clinical models, similar to those reported for rAAV.
Author contributions
CK and DO’C managed the in vivo studies. SI and GD carried out in vitro studies. EG and BS wrote the manuscript. All authors contributed to the article and approved the submitted version.
Data availability
The original contributions presented in the study are included in the article/supplementary material, further inquiries can be directed to the corresponding author.
Ethics statement
The animal study was approved by Charles River Laboratories, accredited by the Association for Assessment and Accreditation of Laboratory Animal Care International (AAALAC). The study was conducted in accordance with the local legislation and institutional requirements.
Funding
The author(s) declare that financial support was received for the research and/or publication of this article. The authors declare that this study received funding from Oxford Biomedica (UK) Limited. The funder was not involved in the study design, collection, analysis, interpretation of data, the writing of this article or the decision to submit it for publication.
Acknowledgments
The authors thank Lucy Barnes, Victoria Kennedy, Robert Powels, Federico Zambon and Melissa Sorci and other members of Oxford Biomedica (United Kingdom) Ltd (OXB) for help data acquisition and analysis and in generating and titrating lentiviral vectors used in these studies.
Conflict of interest
All authors were employees of OxfordBiomedica (UK) Ltd.
Generative AI statement
The authors declare that no Generative AI was used in the creation of this manuscript.
References
1. Bulcha, JT, Wang, Y, Ma, H, Tai, PWL, and Gao, G. Viral vector platforms within the gene therapy landscape. Signal Transduction Targeted Ther (2021) 6:53. doi:10.1038/s41392-021-00487-6
2. Carbonaro, DA, Jin, X, Petersen, D, Wang, X, Dorey, F, Kil, KS, et al. In vivo transduction by intravenous injection of a lentiviral vector expressing human ADA into neonatal ADA gene Knockout mice: a novel form of enzyme replacement therapy for ada deficiency. Mol Ther (2006) 13:1110–20. doi:10.1016/j.ymthe.2006.02.013
3. Carbonaro-Sarracino, DA, Tarantal, AF, Lee, CCI, Kaufman, ML, Wandro, S, Jin, X, et al. Dosing and Re-administration of lentiviral vector for in vivo gene therapy in rhesus monkeys and ADA-deficient mice. Mol Ther - Methods and Clin Development (2020) 16:78–93. doi:10.1016/j.omtm.2019.11.004
4. Dalsgaard, T, Cecchi, CR, Askou, AL, Bak, RO, Andersen, PO, Hougaard, D, et al. Improved lentiviral gene delivery to mouse liver by hydrodynamic vector injection through tail vein. Mol Ther - Nucleic Acids (2018) 12:672–83. doi:10.1016/j.omtn.2018.07.005
5. Brown, BD, Cantore, A, Annoni, A, Sergi, LS, Lombardo, A, Della Valle, P, et al. A microRNA-regulated lentiviral vector mediates stable correction of hemophilia B mice. Blood (2007) 110:4144–52. doi:10.1182/blood-2007-03-078493
6. Milani, M, Canepari, C, Liu, T, Biffi, M, Russo, F, Plati, T, et al. Liver-directed lentiviral gene therapy corrects hemophilia A mice and achieves normal-range factor VIII activity in non-human primates. Nat Commun (2022) 13:2454. doi:10.1038/s41467-022-30102-3
7. Cantore, A, Ranzani, M, Bartholomae, CC, Volpin, M, Valle, PD, Sanvito, F, et al. Liver-directed lentiviral gene therapy in a dog model of hemophilia B. Sci Transl Med (2015) 7:277ra28. doi:10.1126/scitranslmed.aaa1405
8. Nicolas, CT, VanLith, CJ, Hickey, RD, Du, Z, Hillin, LG, Guthman, RM, et al. In vivo lentiviral vector gene therapy to cure hereditary tyrosinemia type 1 and prevent development of precancerous and cancerous lesions. Nat Commun (2022) 13:5012. doi:10.1038/s41467-022-32576-7
9. Berraondo, P, Crettaz, J, Ochoa, L, Pañeda, A, Prieto, J, Trocóniz, IF, et al. Intrahepatic injection of recombinant adeno-associated virus serotype 2 overcomes gender-related differences in liver transduction. Hum Gene Ther (2006) 17:601–10. doi:10.1089/hum.2006.17.601
10. Davidoff, AM, Ng, CYC, Zhou, J, Spence, Y, and Nathwani, AC. Sex significantly influences transduction of murine liver by recombinant adeno-associated viral vectors through an androgen-dependent pathway. Blood (2003) 102:480–8. doi:10.1182/blood-2002-09-2889
11. Mochizuki, S, Mizukami, H, Ogura, T, Kure, S, Ichinohe, A, Kojima, K, et al. Long-term correction of hyperphenylalaninemia by AAV-mediated gene transfer leads to behavioral recovery in phenylketonuria mice. Gene Ther (2004) 11:1081–6. doi:10.1038/sj.gt.3302262
12. Piechnik, M, Amendum, PC, Sawamoto, K, Stapleton, M, Khan, S, Fnu, N, et al. Sex difference leads to differential gene expression patterns and therapeutic efficacy in mucopolysaccharidosis IVA murine model receiving AAV8 gene therapy. Int J Mol Sci (2022) 23:12693. doi:10.3390/IJMS232012693
13. Vigna, E, Amendola, M, Benedicenti, F, Simmons, AD, Follenzi, A, and Naldini, L. Efficient tet-dependent expression of human factor IX in vivo by a new self-regulating lentiviral vector. Mol Ther (2005) 11:763–75. doi:10.1016/j.ymthe.2004.11.017
14. Iqball, S, Beck, DK, Devarajan, G, Khoo, CP, O’Connor, DM, Ellis, S, et al. Lentiviral delivered aflibercept OXB-203 for treatment of neovascular AMD. Mol Ther - Methods and Clin Development (2023) 30:350–66. doi:10.1016/j.omtm.2023.07.001
15. Senova, S, Poupon, C, Dauguet, J, Stewart, HJ, Dugué, GP, Jan, C, et al. Optogenetic Tractography for anatomo-functional characterization of cortico-subcortical neural circuits in non-human primates. Sci Rep (2018) 8:3362. doi:10.1038/s41598-018-21486-8
16. Pañeda, A, Vanrell, L, Mauleon, I, Crettaz, JS, Berraondo, P, Timmermans, EJ, et al. Effect of adeno-associated virus serotype and genomic structure on liver transduction and biodistribution in mice of both genders. Hum Gene Ther (2009) 20:908–17. doi:10.1089/hum.2009.031
17. Shedlovsky, A, McDonald, JD, Symula, D, and Dove, WF. Mouse models of human phenylketonuria. Genetics (1993) 134:1205–10. doi:10.1093/genetics/134.4.1205
18. Balaggan, KS, Binley, K, Esapa, M, Iqball, S, Askham, Z, Kan, O, et al. Stable and efficient intraocular gene transfer using pseudotyped EIAV lentiviral vectors. The J Gene Med (2006) 8:275–85. doi:10.1002/jgm.845
19. Campochiaro, PA, Lauer, AK, Sohn, EH, Mir, TA, Naylor, S, Anderton, MC, et al. Lentiviral vector gene transfer of endostatin/angiostatin for macular degeneration (GEM) study. Hum Gene Ther (2017) 28:99–111. doi:10.1089/hum.2016.117
20. Bell, P, Gao, G, Haskins, ME, Wang, L, Sleeper, M, Wang, H, et al. Evaluation of adeno-associated viral vectors for liver-directed gene transfer in dogs. Hum Gene Ther (2011) 22:985–97. doi:10.1089/hum.2010.194
21. Arruda, V, Scallan, C, Jian, H, Couto, L, Herzog, R, Nichols, T, et al. Comparison of the efficacy on gene transfer by AAV vectors delivered by distinct routes of administration to the liver of hemophilia B dogs. Mol Ther (2004) 9:S40–S41.
22. Kaiser, RA, Weber, ND, Trigueros-Motos, L, Allen, KL, Martinez, M, Cao, W, et al. Use of an adeno-associated virus serotype Anc80 to provide durable cure of phenylketonuria in a mouse model. J Inherit Metab Dis (2021) 44:1369–81. doi:10.1002/jimd.12392
23. Han, SO, Gheorghiu, D, Chang, A, Mapatano, SH, Li, S, Brooks, E, et al. Efficacious androgen hormone administration in combination with adeno-associated virus vector-mediated gene therapy in female mice with pompe disease. Hum Gene Ther (2022) 33:479–91. doi:10.1089/hum.2021.218
24. Han, S, Li, S, McCall, A, Arnson, B, Everitt, JI, Zhang, H, et al. Comparisons of infant and adult mice reveal age effects for liver depot gene therapy in pompe disease. Mol Ther - Methods and Clin Development (2020) 17:133–42. doi:10.1016/j.omtm.2019.11.020
25. Maturana, CJ, Chan, A, Verpeut, JL, and Engel, EA. Local and systemic administration of AAV vectors with alphaherpesvirus latency-associated promoter 2 drives potent transgene expression in mouse liver, kidney, and skeletal muscle. J Virol Methods (2023) 314:114688. doi:10.1016/j.jviromet.2023.114688
26. Bell, P, Wang, L, Chen, S-J, Yu, H, Zhu, Y, Nayal, M, et al. Effects of self-complementarity, codon optimization, transgene, and dose on liver transduction with AAV8. Hum Gene Ther Methods (2016) 27:228–37. doi:10.1089/hgtb.2016.039
27. Wang, L, Wang, H, Morizono, H, Bell, P, Jones, D, Lin, J, et al. Sustained correction of OTC deficiency in spfash mice using optimized self-complementary AAV2/8 vectors. Gene Ther (2012) 19:404–10. doi:10.1038/gt.2011.111
28. Cunningham, SC, Spinoulas, A, Carpenter, KH, Wilcken, B, Kuchel, PW, and Alexander, IE. AAV2/8-mediated correction of OTC deficiency is robust in adult but not neonatal spfash mice. Mol Ther (2009) 17:1340–6. doi:10.1038/mt.2009.88
29. Bell, P, Wang, L, Lebherz, C, Flieder, DB, Bove, MS, Wu, D, et al. No evidence for tumorigenesis of AAV vectors in a large-scale study in mice. Mol Ther (2005) 12:299–306. doi:10.1016/j.ymthe.2005.03.020
30. Kok, CY, Tsurusaki, S, Cabanes-Creus, M, Igoor, S, Rao, R, Skelton, R, et al. Development of new adeno-associated virus capsid variants for targeted gene delivery to human cardiomyocytes. Mol Ther - Methods and Clin Development (2023) 30:459–73. doi:10.1016/j.omtm.2023.08.010
31. Anderson, JM, Arnold, WD, Huang, W, Ray, A, Owendoff, G, and Cao, L. Long-term effects of a fat-directed FGF21 gene therapy in aged female mice. Gene Ther (2023) 31:95–104. doi:10.1038/s41434-023-00422-0
Keywords: lentiviral vectors, adeno-associated virus, AAV, liver, PAH, gender, murine
Citation: Guzman E, Khoo C, O’Connor D, Devarajan G, Iqball S, Souberbielle B, Mitrophanous K and Lad Y (2025) Gender difference in pre-clinical liver-directed gene therapy with lentiviral vectors. Exp. Biol. Med. 250:10422. doi: 10.3389/ebm.2025.10422
Received: 02 November 2024; Accepted: 15 April 2025;
Published: 25 April 2025.
Copyright © 2025 Guzman, Khoo, O’Connor, Devarajan, Iqball, Souberbielle, Mitrophanous and Lad. This is an open-access article distributed under the terms of the Creative Commons Attribution License (CC BY). The use, distribution or reproduction in other forums is permitted, provided the original author(s) and the copyright owner(s) are credited and that the original publication in this journal is cited, in accordance with accepted academic practice. No use, distribution or reproduction is permitted which does not comply with these terms.
*Correspondence: Kyriacos Mitrophanous, ay5taXRyb3BoYW5vdXNAb3hiLmNvbQ==