- 1Chengdu University of Traditional Chinese Medicine, Chengdu, China
- 2Hospital of Chengdu University of Traditional Chinese Medicine, Chengdu, China
Abstract
Observational studies have linked autoimmune diseases (ADs) with rhinosinusitis (RS) manifestations. To establish a causal relationship between ADs and RS, and to explore the potential mediating role of inflammatory mediators between ADs and RS, we utilized Mendelian randomization (MR) analysis. Using a two-sample MR methodology, we examined the causality between multiple sclerosis (MS), rheumatoid arthritis (RA), ankylosing spondylitis (AS), psoriasis (PsO), type 1 diabetes (T1D), Sjogren’s syndrome (SS), celiac disease (CeD), Crohn’s disease (CD), hypothyroidism (HT), Graves’ disease (GD), and Hashimoto’s thyroiditis and their association with chronic and acute rhinosinusitis (CRS and ARS, respectively).To achieve this, we employed three distinct MR techniques: inverse variance weighting (IVW), MR-Egger, and the weighted median method. Our analysis also included a variety of sensitivity assessments, such as Cochran’s Q test, leave-one-out analysis, MR-Egger intercept, and MR-PRESSO, to ensure the robustness of our findings. Additionally, the study explored the role of inflammation proteins as a mediator in these relationships through a comprehensive two-step MR analysis. Among the ADs, MS, RA, T1D, CeD, and HT were determined as risk factors for CRS. Only CeD exhibited a causal relationship with ARS. Subsequent analyses identified interleukin-10 (IL-10) as a potential mediator for the association of MS, RA and HT with CRS, respectively., while C-X-C motif chemokine 10 levels (CXCL10) and T-cell surface glycoprotein CD6 isoform levels (CD6) were found to influence HT’s effect on CRS. Our findings demonstrate a causative link between specific autoimmune diseases and rhinosinusitis, highlighting IL-10, CXCL10, and CD6 as potential mediators in this association.
Impact statement
This study establishes a causal link between several autoimmune diseases (ADs)—specifically multiple sclerosis, rheumatoid arthritis, type 1 diabetes, celiac disease, and hypothyroidism—and rhinosinusitis, employing Mendelian randomization (MR) techniques, including inverse variance weighting, MR-Egger, and weighted median methods, alongside a variety of sensitivity assessments. By demonstrating that several ADs are risk factors for chronic rhinosinusitis and identifying celiac disease as a causal factor for acute rhinosinusitis, this work advances understanding of the interplay between autoimmune disorders and sinusitis. Crucially, it identifies inflammatory mediators such as interleukin-10, C-X-C motif chemokine 10, and CD6 isoform levels as key components in these relationships, offering new insights into potential therapeutic targets. These findings significantly impact the field by providing updated clues of the pathological links between autoimmune conditions and sinus health, with implications for treatment strategies.
Introduction
Autoimmune diseases (ADs), characterized by the immune system’s disarray leading to self-directed tissue and organ damage, include diseases like multiple sclerosis (MS), rheumatoid arthritis (RA), type 1 diabetes (T1D), systemic lupus erythematosus (SLE), celiac disease (CeD), Crohn’s Disease (CD), and autoimmune thyroid diseases (AITD). Their development is tightly linked to genetics, environmental influences, and shifts in the microbiota [1–3]. Epidemiologically, the escalating incidence of ADs is recognized as a significant global health menace, posing a formidable challenge to public health systems [4, 5]. While treatment approaches have evolved, there’s a continuing need for tailored treatment plans and profound investigations into the mechanisms of these complex diseases.
Divided into acute and chronic categories, rhinosinusitis (RS) adversely affects the sinus mucous membranes [6]. The acute rhinosinusitis (ARS) presents with symptoms such as nasal congestion, secretion of mucoid or purulent material, facial discomfort, and olfactory reduction, not exceeding 4 weeks in duration [6, 7]. Chronic rhinosinusitis (CRS), lasting over 12 weeks, invariably involves nasal inflammation, often with preceding rhinitis symptoms [6, 8]. Its occurrence is tightly linked with factors including bacterial, viral, genetic, immune deficiency, environmental components, and air quality [8, 9], with nasal polyps and turbinate enlargement also contributing to the severity [10, 11]. Management options range from antibiotics, vasoconstrictors, antihistamines, and hypertonic saline for symptomatic relief to surgical procedures, with treatment efficacy potentially influenced by factors such as weather and emotional states [9, 12].
Inflammation is a key player in the progression of diseases, holding significant importance for both science and public health. Triggered by bacteria such as Streptococcus pneumoniae and viruses like coronaviruses, ARS involves the activation of various inflammatory cytokines, including IFN-α, IL-1β, and IL-6 [13, 14]. CRS, mirroring the complexity of chronic diseases, poses a significant challenge in finding a cure. The expression and regulation of cytokines are paramount in its etiology. CRS inflammation is differentiated into three categories based on the elevation of specific lymphocyte cytokines: Types 1, 2, and 3 [15, 16]. The complexity of interactions between immune cells and cytokines plays a critical role in CRS-related inflammation and tissue remodeling [17]. As mediators of communication among immune cells, cytokines are crucial in the regulation of immune responses and inflammation [18]. Aberrant cytokine levels in autoimmune diseases can result in sustained inflammation, leading to tissue damage and advancement of the disease [18, 19]. It has been indicated that autoimmune diseases could heighten the risk of CRS, influencing its prognosis [20, 21]. While the direct causal link remains to be established, the association between immune dysfunctions and inflammatory responses directs future research towards understanding the interplay between these conditions and enhancing therapeutic approaches.
Mendelian randomization (MR) utilizes genetic variants as instruments to investigate the causal relationships between particular exposures and outcomes, leveraging single nucleotide polymorphisms (SNPs) from genome-wide association studies (GWAS) to bypass traditional study confounders and enhance causal inference accuracy. This study is centered on exploring the Mendelian causality between ADs and RS, specifically focusing on the role of inflammatory mediators. Employing large GWAS datasets from European populations, through dual-sample MR analysis and a two-phase mediation approach, our aim is to reveal both the direct and indirect causal links between ADs and RS, contributing to a deeper biological understanding of their mutual influences.
Materials and methods
Study design
To delineate the causal dynamics and possible mediating roles between ADs and RS, chronic and acute, this study embarked on a two-phased Mendelian randomization approach. The initial phase employed univariable MR (UVMR) to explore the causal relationships between ADs and RS. Only exposures with a significant causal link to RS, and where reverse causation was excluded, were subjected to further scrutiny. The subsequent phase was dedicated to identifying and measuring the influence of circulating inflammatory proteins as mediators in the causal pathway from ADs to RS. Conducted in accordance with the “Strengthening the Reporting of Observational Studies in Epidemiology Using Mendelian Randomization,” this investigation required no additional ethical approvals or informed consent, leveraging publicly accessible GWAS data. The design and analytical procedure are summarized in Figure 1 (All references in the Methods section are listed in Supplementary Table S1).
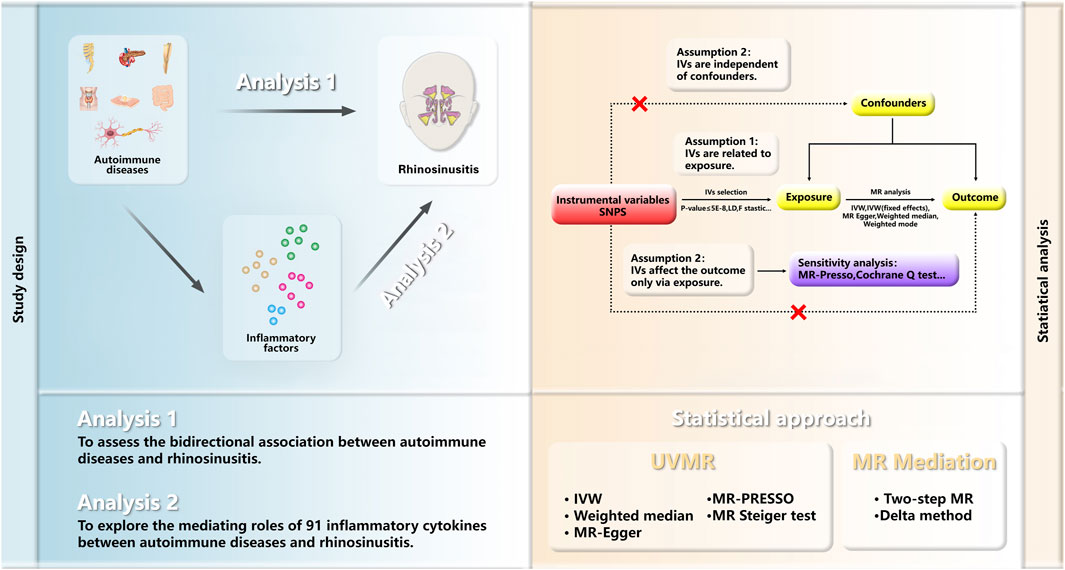
Figure 1. Overview of the MR Study Design. The study is structured into two analytical phases. Analysis 1 employed UVMR to investigate the causal links between ADs and RS. Analysis 2 utilized a two-step MR approach to explore the potential mediation effect of inflammatory proteins on the causal association between ADs and RS. MR, Mendelian randomization; UVMR, univariable Mendelian randomization analyses; IVs, Instrumental variables; SNPs, single-nucleotide polymorphisms; LD, linkage disequilibrium; WM, weighted median; IVW, inverse variance weighted; PRESSO pleiotropy residual sum and outlier.
Data sources
Employing publicly accessible GWAS summary datasets, this MR study assessed designated traits (refer to Table 1). For T1D, genetic tools were sourced from a meta-analysis involving UK and Sardinian participants (7,467 cases versus 10,218 controls). The RA dataset originated from a meta-analysis involving 14,361 cases and 43,923 controls, while genetic instrument variables (IVs) for MS were furnished by a German study (4,888 cases and 10,395 controls). Data for Ankylosing spondylitis (AS) were contributed by the International Genetics of Ankylosing Spondylitis Consortium (IGAS) (9,069 cases and 13,578 controls), and IVs for Psoriasis (PsO) came from research involving 15,967 cases and 28,194 controls. Systemic lupus erythematosus (SLE) data involved 5,201 European descent cases and 9,066 controls. The IEU GWAS database provided information for Sjogren’s syndrome (407,746 samples), Celiac disease (11,812 cases and 11,837 controls), Hypothyroidism or myxoedema (405,357 samples), and Crohn’s disease (17,897 cases and 33,977 controls). Graves’ disease (1,678 cases and 456,942 controls) and Hashimoto thyroiditis (15,654 cases and 379,986 controls) data were derived from a meta-analysis of the European population.
Data regarding rhinosinusitis cases were retrieved from the FinnGen project’s R10 dataset, involving 412,181 individuals with European descent, documenting 21,311,942 variants. Summary GWAS results for ARS (with 10,916 cases against 182,945 controls) and CRS (8,524 cases compared to 167,849 controls) were acquired from FinnGen1. Information on circulating inflammatory proteins, considered as potential mediators, was taken from a recent European study spanning 11 cohorts, which analyzed 91 plasma proteins across 14,824 samples and performed a comprehensive genome-wide protein quantitative trait loci (pQTL) study. Detailed data on the inflammatory proteins can be found in Supplementary Table S2.
Selection criteria for IVs
When selecting appropriate genetic IVs from GWAS of 12 ADs using the TwoSampleMR package in R studio (default settings), we primarily chose SNPs with p-values less than 5.0E-08 to ensure genome-wide significance. Specifically, for Sjogren’s syndrome (SS), due to a lack of SNPs meeting the p < 5.0E-08 criterion, we lowered the threshold to p < 5.0E-06 to secure a sufficient number of valid IVs. MR-Steiger analysis was utilized to assess the direction and validity of causal relationships, with p > 0.05 indicating potential reverse causation, prompting reverse MR analysis. Lower significance threshold to consider p < 5.0E-06 was also adopted when analyzing the potential mediating effects of inflammatory proteins. Independence of SNPs was ensured using an r2 = 0.001 and a clumping window of 10,000 kb. The validity of all IVs was evaluated based on the F-statistic (F = β^2/se^2), considering IVs effective when F values exceeded 10.
Statistical analysis
Univariable Mendelian randomization
A UVMR approach was used to examine causal links between ADs and RS, including inverse-variance weighted (IVW), weighted median, and MR-Egger analysis. The IVW method, serving as the main approach, offers precise causal estimates under the condition that all IVs are valid. MR-Egger method, which offers adjustments for estimate heterogeneity and an intercept term for evaluating pleiotropy, enhances the robustness of causal inference. The weighted median approach, effective when a majority of SNPs are credible IVs, allows for an unbiased estimation of causality. Additionally, MR-Steiger analysis was utilized to verify causal direction and mitigate reverse causality concerns among SNPs.
Mediation analysis
After conducting UVMR to assess causal links between ADs and RS, significant causations were selected for mediation effect analysis in a two-step approach. The research delved into the potential mediating roles of 91 inflammatory proteins between ADs and RS. The total causal effect of ADs on RS (β) was first identified via UVMR, followed by an evaluation of the causal influences of ADs on 91 inflammatory proteins (β1) and their subsequent effects on RS (β2). Diseases with evidence of reverse causality were excluded from the mediation analysis. The correlation between inflammatory proteins and the risk for ADs or RS was assessed via two step MR analyses, using the IVW method with p < 0.05 indicating statistical significance. For inflammatory proteins related to both ADs and RS, the mediation effect was examined, calculated as the product of β1 and β2 divided by β, and the proportion of mediation effect was estimated using the delta method for 95% confidence intervals (CI).
Sensitivity analysis
This research applied MR-Egger and weighted median methods for sensitivity analysis to confirm the UVMR analysis’s robustness, additionally utilizing the Cochran Q test to examine IVs heterogeneity. The MR-Egger intercept was deployed to evaluate pleiotropy, depicted in scatter diagrams. High-heterogeneity SNPs were removed through a leave-one-out procedure, with a subsequent reassessment of outcomes. MR-Presso identified and eliminated outlier SNPs, enhancing estimation accuracy. MR-Steiger testing further affirmed causal direction and reliability. The analyses utilized R software (version 4.0.2), specifically through the “TwoSampleMR” (version 0.5.8), “MendelianRandomization” (version 0.9.0), and “MRPRESSO” packages, as detailed in Supplementary Table S1.
Results
Effects of ADs on RS
The MR results for the impact of ADs on CRS are detailed in Figure 2. After rigorous selection and exclusion, the IVs demonstrated F-statistics greater than 10, indicating no weak instrument bias (Supplementary Table S3). If significant heterogeneity was detected (Cochrane Q test p-value <0.05), the random effects model of the IVW method was employed as the primary analytical approach; otherwise, the fixed effects model of IVW was utilized. Two-sample MR (TSMR) revealed that the genetic predisposition to CRS is significantly associated with MS (OR = 1.04, 95% CI: 1.01-1.07, p = 1.51E-02), CeD (OR = 1.04, 95% CI: 1.01-1.06, p = 1.42E-03), RA (OR = 1.05, 95% CI: 1.01-1.09, p = 2.72E-02), T1D (OR = 1.04, 95% CI: 1.02-1.06, p = 8.67E-05), and hypothyroidism or myxedema (OR = 1.07, 95% CI: 1.02-1.12, p = 6.36E-03). The MR-Egger intercept analysis did not demonstrate horizontal pleiotropy, and MR-Steiger testing validated the directionality of our causal inferences (Supplementary Table S4). Scatter plots, funnel plots, and leave-one-out analyses further describe the results (Supplementary Figures S1–12). Similarly, using two-sample MR and the IVW method, only the relationship between CeD and ARS was significant (OR = 1.04, 95% CI: 1.01-1.06, p = 2.07E-03). The MR results for ADs on ARS are presented in Supplementary Figure S13, with sensitivity analyses and MR-Steiger test results in Supplementary Table S4. Scatter plots, funnel plots, and leave-one-out analyses further detail these findings (Supplementary Figures S14–25).
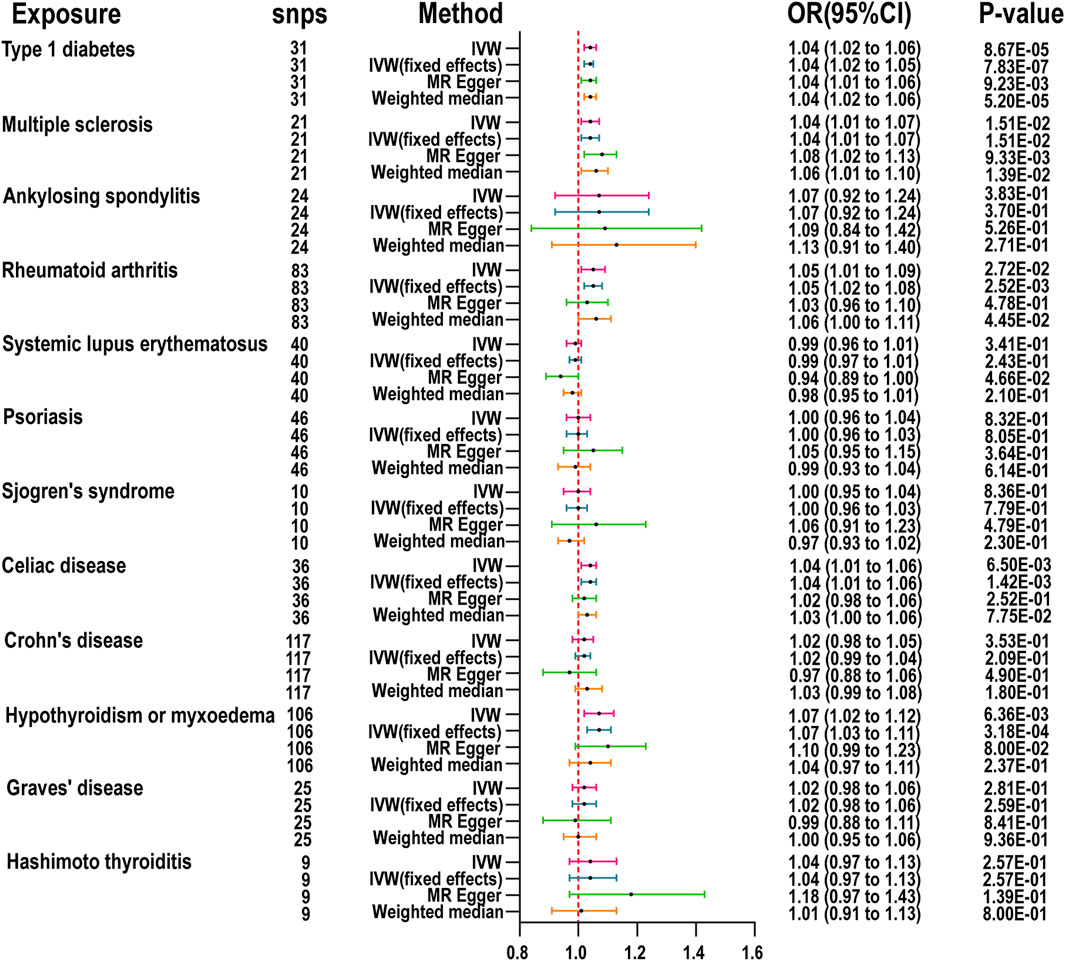
Figure 2. MR is used to examine the link between 12 autoimmune diseases and chronic rhinosinusitis. inverse variance weighted (IVW), number of SNPs used in MR (nsnps), odds ratio (OR), confidence interval (Cl).
Mediating effect of inflammatory proteins
Following the MR analysis between ADs and RS, we identified five ADs with a close relationship to RS and compared them against 91 circulating inflammatory proteins. Analysis using TSMR and the IVW method revealed that 15, 10, 9, 7, and 12 inflammatory proteins were significantly associated with MS, CeD, RA, T1D, and hypothyroidism (HT), respectively (Figure 3). To investigate which specific inflammatory proteins could serve as potential mediators in the causal relationship between ADs and RS, we analyzed those proteins significantly associated with ADs as new exposures in relation to RS. The MR-Steiger test was employed to validate the direction of causality, excluding reverse causal associations from mediation analysis. After SNP filtering and MR analysis, only inflammatory proteins significantly related to both ADs and RS were considered for mediation analysis. The IVW method indicated that interleukin-10 levels (IL-10) were significantly associated with MS (OR = 0.97, 95% CI: 0.95–0.99, p = 4.04E-03), RA (OR = 1.04, 95% CI: 1.01–1.06, p = 1.13E-03), and HT (OR = 1.05, 95% CI: 1.02–1.08, p = 3.87E-04), as detailed in Figure 4. Additionally, T-cell surface glycoprotein CD6 isoform levels (CD6) were significantly related to HT (OR = 1.03, 95% CI: 1.00–1.06, p = 4.92E-02) and CRS (OR = 1.16, 95% CI: 1.03–1.30, p = 1.16E-02), and C-X-C motif chemokine 10 levels (CXCL10) to HT (OR = 1.05, 95% CI: 1.02–1.08, p = 1.90E-03) and CRS (OR = 1.20, 95% CI: 1.06–1.34, p = 2.74E-03), acting as mediators. Weighted median and MR-Egger analyses confirmed the IVW, with heterogeneity and pleiotropy detailed in Supplementary Table S5. Scatter plots, leave-one-out plots, and funnel plots for the relevant instrumental variables provided further evidence (Supplementary Figures S26–S33).
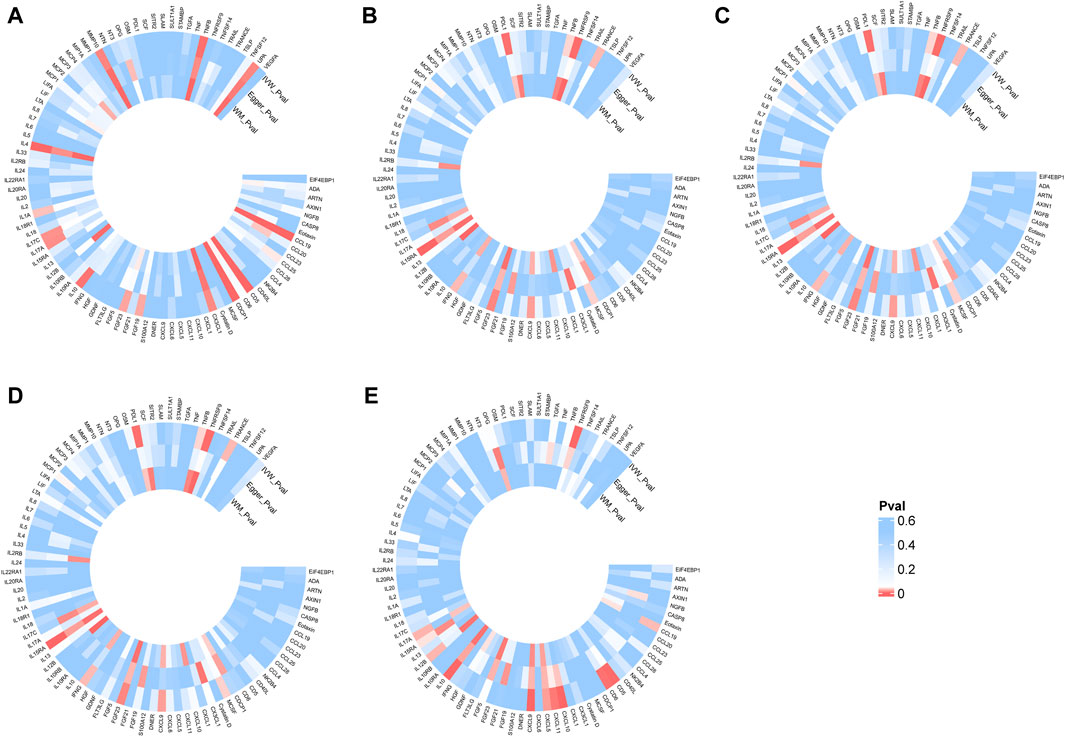
Figure 3. Heatmap of the causal relationships between five autoimmune diseases and 91 inflammatory proteins. (A): Effects of multiple sclerosis on inflammatory proteins. (B): Effects of celiac disease on inflammatory proteins. (C): Effects of type 1 diabetes on inflammatory proteins; (D), Effects of rheumatoid arthritis on inflammatory proteins. (E): Effects of hypothyroidism or myxedema on inflammatory proteins. The color gradient from blue to red indicates an increasing significance of the causal relationship.inverse variance weighted (IVW), number of SNPs used in MR (nsnps), odds ratio (OR), confidence interval (Cl).
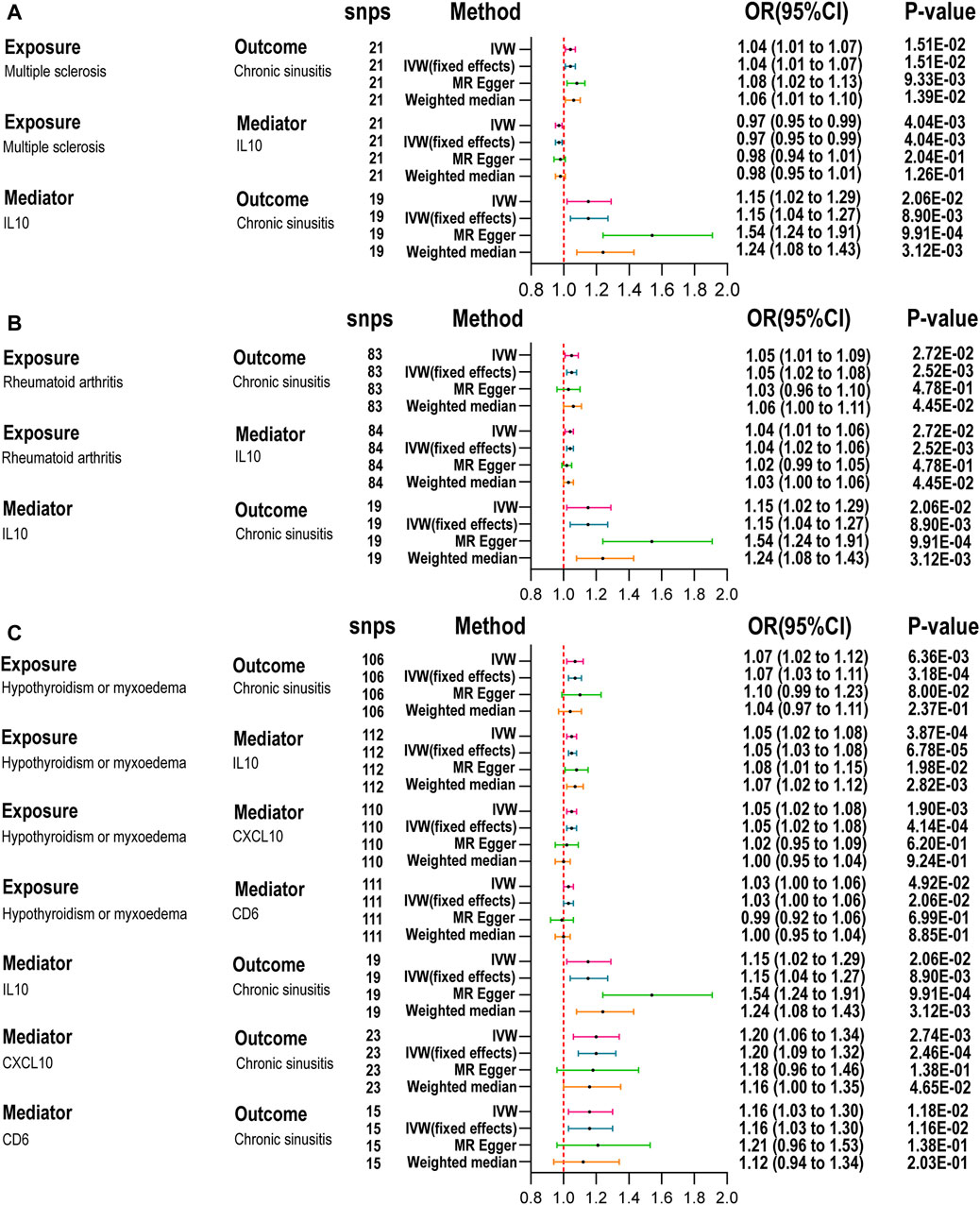
Figure 4. Mediation analysis of the effect of autoimmune diseases on chronic rhinosinusitis via inflammatory proteins. (A): Summary MR for the effect of MS on CRS via inflammatory factor. (B): Summary MR for the effect of RA on CRS via inflammatory factor. (C): Summary MR for the effect of HT on CRS via inflammatory factor; inverse variance weighted (IVW), number of SNPs used in MR (nsnps), odds ratio (OR), confidence interval (Cl) Table 2. IL-10, nterleukin-10; CXCL10, C-X-C motif chemokine 10; CD6, T-cell surface glycoprotein CD6 isoform.
We further quantified the mediating effects of these inflammatory proteins (Table 2), finding IL-10 levels mediated the causal relationship between MS, RA, and HT with CRS. Specifically, the mediation effects were −11.65% (95% CI: −26.73 to 3.44) for MS, 10.73% (95% CI: −3.30–24.76) for RA, and 10.48% (95% CI: −1.84–22.79) for HT. For HT and CRS, the mediation proportions for CXCL10 and CD6 were 12.27% (95% CI: −1.95–26.48) and 6.59% (95% CI: −2.99–16.17), respectively. No inflammatory proteins were identified as mediators between ADs and ARS (Supplementary Figure S34).
Discussion
Employing multiple GWAS datasets, this study leverages Mendelian randomization to shed light on the causal links between ADs and RS. Our genetic analyses identified causal associations between five ADs and CRS: MS, CeD, RA, T1D, and HT. The relationship between CeD and ARS is significant. The role of cytokines has been increasingly recognized in the etiology and progression of ADs and RS, though their exact mechanism within the causal framework between ADs and RS is not fully understood. Given the increased risk of developing RS associated with these ADs, we further employed a large-scale genetic dataset comprising 91 cytokines to explore their role in these relationships.
Evidence suggests a notable link between ADs and CRS, indicating that ADs could serve as risk factors for CRS. A cross-sectional analysis highlighted the disparity in CRS prevalence among various ADs, including multiple sclerosis at 1.4%, ankylosing spondylitis at 6.05%, systemic lupus erythematosus at 3.9%, rheumatoid arthritis at 5.0%, psoriasis at 3.8%, and inflammatory bowel disease at 3.5%. Additionally, this study identified the prevalence of CRS with nasal polyps (CRSwNP) at 9.3% and CRS without nasal polyps (CRSsNP) at 91.7%, analyzing the proportions of CRSwNP and CRSsNP within these ADs [31]. A retrospective study in Asia confirmed the significant correlation between CRS and various ADs, particularly in ankylosing spondylitis, SLE, psoriasis, RA, and Sjögren's syndrome [32]. Our research indicates that, beyond MS and RA, T1D, CeD, and HT are also significantly associated with CRS. It is well recognized that immune dysfunction in diabetes mellitus (DM) patients facilitates infections. A retrospective cohort study of 376 CRS patients found that those with DM were prone to Gram-negative bacterial sinus infections [33]. DM patients are at high risk for mucormycosis, susceptible to severe rhino-orbital-cerebral and pulmonary infections. Hence, prompt diagnosis and management of potential life-threatening fungal infections are crucial for DM patients [22, 23, 34]. Although CeD, as a multisystem autoimmune disease, may cause extraintestinal otolaryngological manifestations [24, 25], current research is insufficient to clarify this relationship, indicating the need for further investigation. Limited studies exist on the relationship between CRS and HT. Existing research has shown that patients with HT experience prolonged mucociliary clearance times [26, 27], and animal experiments have demonstrated nasal mucosal hypertrophy, cilia loss, and inflammation following thyroidectomy [28]. These findings align with our results, suggesting the importance of close monitoring for respiratory diseases and sinus infections in patients with HT.
The association between inflammatory proteins and ADs involves intricate regulation of the immune system and a disruption in self-tolerance. Studies have indicated that the expression of tumor necrosis factor-alpha (TNF-α), interferon-gamma (IFN-γ), and several interleukins (such as IL-1, IL-6, IL-12, and IL-17) is elevated in autoimmune diseases, driving inflammation and tissue damage [18]. These cytokines activate immune cells, leading to self-tissue destruction and disease progression [19]. Concurrently, regulatory cytokines, such as IL-10, IL-4, and TGF-β, suppress immune responses by promoting immune tolerance and limiting inflammatory reactions, thereby protecting tissues [18]. Rhinosinusitis, involving inflammation of the nasal sinus mucosa, can be triggered by infections, allergies, or environmental factors, with the disease mechanism centrally involving the modulation of immune system responses by inflammatory proteins [29].
When examining inflammatory proteins as mediators between ADs and RS, we identified IL-10 as a mediator in the relationships between MS, RA, and HT with CRS. As a key anti-inflammatory cytokine, IL-10 effectively suppresses the production of pro-inflammatory cytokines, including TNF-α and IL-1, by various immune cells, such as macrophages and dendritic cells. It also enhances the development of regulatory T cells, regulating immune reactions accordingly [30, 35]. In MS patients, serum IL-10 secretion decreases before MS relapse and increases during remission [36], partially aligning with our findings of reduced IL-10 levels in MS patients. In animal models of autoimmune encephalomyelitis (EAE), IL-10 deficiency resulted in more severe disease progression [37, 38]. Studies have shown increased concentrations of IL-10 in the serum and synovial fluid of RA patients [39, 40]. In animal studies, IL-10 reduced symptoms of RA in rats, curbed neutrophil penetration and activation within synovial tissues, and diminished the production of pro-inflammatory cytokines such as TNF, IL-1, and IL-6 [41, 42]. These findings and our results underscore IL-10’s undisputed regulatory function as an anti-inflammatory cytokine in arthritis. In patients with autoimmune thyroid disease (AITD), IL-10 mRNA expression was found to increase significantly, decreasing as the autoimmune process subsided [43]. IL-10 mitigates inflammation in early thyroiditis by inhibiting Th1 cytokines like TNF-α and IL-1, while in later stages, Th2 cell activation promotes humoral responses [44, 45]. Changes in IL-10 levels among CRS patients remain contentious [46–49]. Moreover, viral and bacterial infections play a crucial role in exacerbating CRS, where IL-10 demonstrates potential therapeutic value in regulating inflammation and ADs by modulating M2 macrophages and mitigating bacterial inflammatory responses triggered by Toll-like receptor signaling [29, 50, 51]. Therefore, these immunoregulatory functions of IL-10 suggest its potential therapeutic role in preventing inflammatory and autoimmune diseases.
The IL-10 cytokine family, which includes IL-19, IL-20, IL-22, IL-24, and IL-26, is integral to regulating anti-inflammatory, pro-inflammatory, and antiviral responses. Although they were not found to act as mediators in our study, they remain key targets for immunomodulatory therapy [52]. Elevated IL-19 expression has been observed in the mononuclear cells within the synovial fluid of RA sufferers [53]. Antibodies targeting IL-19 have prevented arthritis and osteolysis in animal models by diminishing the secretion of pro-inflammatory cytokines, including TNF-α, IL-1β, IL-6, and RANKL [54]. In psoriasis (PsO), elevated levels of IL-19 have been observed, which induce dysplasia and activation of keratinocytes [55, 56], and also prompts CD8+ T cells to generate Keratinocyte Growth Factor [57]. IL-20 is linked with increased inflammatory activity in RA joints, and its antagonists may offer therapeutic benefits [58]; its overexpression causes psoriasis-like skin conditions [59], while blocking its pathway reduces psoriasis symptoms [60]. IL-22 is enhanced during active MS, affecting T cell function and participating in the disease process [61], and by regulating keratinocyte differentiation, it influences the mechanisms of PsO [62]. IL-24 aggravates inflammation in RA and PsO, yet it plays a protective role in inflammatory bowel disease by sustaining epithelial and mucosal integrity, thus displaying a bidirectional regulatory capacity in inflammation [63]. In summary, through their complex pro-inflammatory and anti-inflammatory functions, members of the IL-10 family play a crucial regulatory role in ADs.
Our investigation also reveals a causal connection between CXCL10 and the relationship between HT and CRS, highlighting CXCL10’s critical function in immune cell migration to areas of inflammation or damage, thus contributing to the progression of ADs. Elevated CXCL10 expression in conditions such as RA and AITD is closely associated with disease severity and inflammation levels [64, 65]. By interacting with its receptor, CXCR3, CXCL10 triggers Th1 immune responses, promoting infiltration by inflammatory cells and increasing the production of inflammatory mediators, which aggravates tissue damage [64, 66]. Moreover, airway epithelial cells increase the secretion of inflammatory mediators such as IL-1, IL-6, IL-8, and CXCL10 following infections [67, 68], recruiting inflammatory cells to the inflammation site and intensifying the response [69]. Thus, CXCL10, and its receptor CXCR3, are pivotal in enhancing immune responses and inflammation, presenting new avenues for therapeutic intervention in inflammatory diseases and ADs. Additionally, our study identified CD6 as a significant mediator. Primarily found on T and some B cells, CD6 is integral to the immunoglobulin superfamily [70]. It significantly influences T cell activation by interacting with its ligand ALCAM (CD166) [71], bolstering TCR signal transmission and encouraging T cell activation and proliferation [70]. CD6’s role in immune cell adhesion and migration is vital for inflammation and immune surveillance. With CD6 anomalously expressed in multiple ADs, including MS, SLE, and RA [72], its dysregulated activity could exacerbate inflammatory symptoms and tissue damage. Despite limited direct research on CD6 in HT and CRS, its role in autoimmune processes suggests potential underlying connections that merit further exploration.
Beyond the aforementioned mediatory inflammatory proteins, the significance of other inflammatory proteins in ADs should not be overlooked. For instance, IL-17 levels are elevated in patients with MS, and its inhibitors can mitigate brain damage, underscoring the role of IL-17 in promoting inflammation in the nervous system and exacerbating demyelination and axonal damage [73]. Synovitis in RA is characterized by the continuous influx of immune cells and the production of various pro-inflammatory cytokines such as TNF, IL-1, IL-17, and IL-22, which stimulate bone and cartilage inflammation and damage [74]. In T1D, IL-10, TGF-β, and IL-33 aid in reinstating immune tolerance and shielding β-cells from harm, whereas IL-6, IL-17, IL-21, and TNF accelerate the disease’s advancement [75]. Th17 cells, along with their associated inflammatory cytokines IL-17 and IL-23, play a role in the development of autoimmune thyroid (AITD) [44]. IL-15 levels correlate positively with active lesions in patients with celiac disease (CeD), highlighting its importance in CeD [76]. In multiple sclerosis, CC chemokines such as CCL2 and CCL5 promote central nervous system inflammation by regulating immune cell migration [77]. In autoimmune diseases, fibroblast growth factors (FGFs) and their receptors play key roles in inflammatory responses and tissue repair, such as FGF-23, which promotes pro-inflammatory responses of M1 macrophages during infections [78].
Through the application of Mendelian randomization, this research has adeptly navigated confounders and the issue of reverse causation, achieving credible causal effect estimations from observational data [79]. The use of mixed data MR models, incorporating substantial GWAS datasets, has notably increased the efficiency of the analysis compared to smaller-scale, single data models [80]. The study, however, faces significant limitations, notably its sample composed entirely of European individuals, which narrows the generalizability of its findings, and the persistent heterogeneity that could not be fully eradicated, despite thorough attempts with multiple sensitivity analyses, leaving a risk of diversity. Certain Mendelian randomization findings lack empirical backing and thus necessitate careful interpretation, yet they provide a logical foundation for further research. Summarily, the MR analysis elucidated the causal connections between autoimmune diseases and rhinosinusitis, and the mediating influence of inflammatory proteins between ADs and CRS, offering essential biomarkers and potential targets for deciphering disease mechanisms and crafting therapeutic strategies.
Author contributions
YL: Conceptualization, Methodology, Resources, Writing–original draft. SY: Formal Analysis, Data curation, Writing–review and editing. XC: Data curation, Validation, Writing–review and editing. CL: Software, Visualization, Writing–review and editing. QC: Project administration, Supervision, Writing–review and editing.
Data availability
The original contributions presented in the study are included in the article/Supplementary Material, further inquiries can be directed to the corresponding author.
Ethics statement
The data used in this study are derived from publicly available aggregated data, and the ethical approval for each individual study can be found in the original publications, thus no additional ethical approval is required.
Funding
The author(s) declare that no financial support was received for the research, authorship, and/or publication of this article.
Conflict of interest
The authors declare that the research was conducted in the absence of any commercial or financial relationships that could be construed as a potential conflict of interest.
Supplementary material
The Supplementary Material for this article can be found online at: https://www.ebm-journal.org/articles/10.3389/ebm.2024.10196/full#supplementary-material
Footnotes
References
1. Rose, NR. Prediction and prevention of autoimmune disease in the 21st century: a review and preview. Am J Epidemiol (2016) 183(5):403–6. doi:10.1093/aje/kwv292
2. Jörg, S, Grohme, DA, Erzler, M, Binsfeld, M, Haghikia, A, Müller, DN, et al. Environmental factors in autoimmune diseases and their role in multiple sclerosis. Cell Mol Life Sci (2016) 73(24):4611–22. doi:10.1007/s00018-016-2311-1
3. Chen, B, Sun, L, and Zhang, X. Integration of microbiome and epigenome to decipher the pathogenesis of autoimmune diseases. J Autoimmun (2017) 83:8331–42. doi:10.1016/j.jaut.2017.03.009
4. Ngo, ST, Steyn, FJ, and Mccombe, PA. Gender differences in autoimmune disease. Front Neuroendocrinology (2014) 35(3):347–69. doi:10.1016/j.yfrne.2014.04.004
5. Conrad, N, Verbeke, G, Molenberghs, G, Goetschalckx, L, Callender, T, Cambridge, G, et al. Autoimmune diseases and cardiovascular risk: a population-based study on 19 autoimmune diseases and 12 cardiovascular diseases in 22 million individuals in the UK. The Lancet (2022) 400(10354):733–43. doi:10.1016/S0140-6736(22)01349-6
6. Bleier, BS, and Paz-Lansberg, M. Acute and chronic sinusitis. Med Clin North America (2021) 105(5):859–70. doi:10.1016/j.mcna.2021.05.008
7. Meltzer, EO, Hamilos, DL, Hadley, JA, Lanza, DC, Marple, BF, Nicklas, RA, et al. Rhinosinusitis: establishing definitions for clinical research and patient care. Otolaryngology--Head Neck Surg (2004) 131(6 Suppl. l):S1–62. doi:10.1016/j.otohns.2004.09.067
8. Benninger, MS, Ferguson, BJ, Hadley, JA, Hamilos, DL, Jacobs, M, Kennedy, DW, et al. Adult chronic rhinosinusitis: definitions, diagnosis, epidemiology, and pathophysiology. Otolaryngology-Head Neck Surg (2003) 129(3 Suppl. l):S1–32. doi:10.1016/s0194-5998(03)01397-4
9. Rosenfeld, RM, Piccirillo, JF, Chandrasekhar, SS, Brook, I, Ashok Kumar, K, Kramper, M, et al. Clinical practice guideline (update): adult sinusitis. Otolaryngology-Head Neck Surg (2015) 152(2 Suppl. l):S1–39. doi:10.1177/0194599815572097
10. Fokkens, WJ, Lund, VJ, Hopkins, C, Hellings, PW, Kern, R, Reitsma, S, et al. European position paper on rhinosinusitis and nasal polyps 2020. Rhinology J (2020) 0(Suppl. S29):1–464. doi:10.4193/Rhin20.600
11. Alsowey, AM, Abdulmonaem, G, Elsammak, A, and Fouad, Y. Diagnostic performance of multidetector computed tomography (mdct) in diagnosis of sinus variations. Pol J Radiol (2018) 82:82713–725. doi:10.12659/PJR.903684
12. Orlandi, RR, Kingdom, TT, Smith, TL, Bleier, B, Deconde, A, Luong, AU, et al. International consensus statement on allergy and rhinology: rhinosinusitis 2021. Int Forum Allergy Rhinol (2021) 11(3):213–739. doi:10.1002/alr.22741
13. Fokkens, WJ, Lund, VJ, Mullol, J, Bachert, C, Alobid, I, Baroody, F, et al. Epos 2012: european position paper on rhinosinusitis and nasal polyps 2012. A summary for otorhinolaryngologists. Rhinology J (2012) 50(1):1–12. doi:10.4193/Rhino12.000
14. Riechelmann, H, Deutschle, T, Rozsasi, A, Keck, T, Polzehl, D, and Bürner, H. Nasal biomarker profiles in acute and chronic rhinosinusitis. Clin Exp Allergy (2005) 35(9):1186–91. doi:10.1111/j.1365-2222.2005.02316.x
15. Stevens, WW, Peters, AT, Tan, BK, Klingler, AI, Poposki, JA, Hulse, KE, et al. Associations between inflammatory endotypes and clinical presentations in chronic rhinosinusitis. J Allergy Clin Immunol Pract (2019) 7(8):2812–20.e3. doi:10.1016/j.jaip.2019.05.009
16. Samitas, K, Carter, A, Kariyawasam, HH, and Xanthou, G. Upper and lower airway remodelling mechanisms in asthma, allergic rhinitis and chronic rhinosinusitis: the one airway concept revisited. Allergy (2018) 73(5):993–1002. doi:10.1111/all.13373
17. Gong, X, Han, Z, Fan, H, Wu, Y, He, Y, Fu, Y, et al. The interplay of inflammation and remodeling in the pathogenesis of chronic rhinosinusitis: current understanding and future directions. Front Immunol (2023) 14:141238673. doi:10.3389/fimmu.2023.1238673
18. O'Shea, JJ, Ma, A, and Lipsky, P. Cytokines and autoimmunity. Nat Rev Immunol (2002) 2(1):37–45. doi:10.1038/nri702
19. Yadav, D, and Sarvetnick, N. Cytokines and autoimmunity: redundancy defines their complex nature. Curr Opin Immunol (2003) 15(6):697–703. doi:10.1016/j.coi.2003.09.006
20. Kwah, JH, Somani, SN, Stevens, WW, Kern, RC, Smith, SS, Welch, KC, et al. Clinical factors associated with acute exacerbations of chronic rhinosinusitis. J Allergy Clin Immunol (2020) 145(6):1598–605. doi:10.1016/j.jaci.2020.01.023
21. Min, J, and Tan, BK. Risk factors for chronic rhinosinusitis. Curr Opin Allergy Clin Immunol (2015) 15(1):1–13. doi:10.1097/ACI.0000000000000128
22. Khoshbayan, A, Didehdar, M, Chegini, Z, Taheri, F, and Shariati, A. A closer look at pathogenesis of cerebral mucormycosis in diabetic condition: a mini review. J Basic Microbiol (2021) 61(3):212–8. doi:10.1002/jobm.202000692
23. Kashyap, S, Bernstein, J, Ghanchi, H, Bowen, I, and Cortez, V. Diagnosis of rhinocerebral mucormycosis by treatment of cavernous right internal carotid artery occlusion with mechanical thrombectomy: special case presentation and literature review. Front Neurol (2019) 10:10264. doi:10.3389/fneur.2019.00264
24. Medina-Banegas, A, Pastor-Quirante, FA, Osete-Albaladejo, J, López-Meseguer, E, and López-Andreu, F. Nasal septal perforation in a patient with subclinical celiac disease: a possible new association. Eur Arch Otorhinolaryngol (2005) 262(11):928–31. doi:10.1007/s00405-004-0865-2
25. Mohn, A, di Ricco, L, Magnelli, A, and Chiarelli, F. Celiac disease--associated vertigo and nystagmus. J Pediatr Gastroenterol Nutr (2002) 34(3):317–8. doi:10.1097/00005176-200203000-00019
26. Kulekci Ozturk, S, Sakci, E, and Kavvasoglu, C. Rhinitis in patients with acquired hypothyroidism. Eur Arch Otorhinolaryngol (2021) 278(1):87–92. doi:10.1007/s00405-020-06254-7
27. Uysal, IO, Gökakın, AK, Karakuş, CF, Deveci, K, Hasbek, Z, and Sancakdar, E. Evaluation of nasal mucociliary activity in iatrogenic hypothyroidism. Eur Arch Otorhinolaryngol (2013) 270(12):3075–8. doi:10.1007/s00405-013-2439-7
28. Proud, GO, and Lange, RD. The effect of thyroidectomy on the nasal mucosa of experimental animals. The Laryngoscope (1957) 67(3):201–7. doi:10.1288/00005537-195703000-00003
29. Tan, KS, Yan, Y, Ong, HH, Chow, VTK, Shi, L, and Wang, D. Impact of respiratory virus infections in exacerbation of acute and chronic rhinosinusitis. Curr Allergy Asthma Rep (2017) 17(4):24. doi:10.1007/s11882-017-0693-2
30. Saraiva, M, Vieira, P, and O'Garra, A. Biology and therapeutic potential of interleukin-10. J Exp Med (2020) 217(1):e20190418. doi:10.1084/jem.20190418
31. Chandra, RK, Lin, D, Tan, B, Tudor, RS, Conley, DB, Peters, AT, et al. Chronic rhinosinusitis in the setting of other chronic inflammatory diseases. Am J Otolaryngol (2011) 32(5):388–91. doi:10.1016/j.amjoto.2010.07.013
32. Shih, L, Hsieh, H, Tsay, GJ, Lee, IT, Tsou, Y, Lin, C, et al. Chronic rhinosinusitis and premorbid autoimmune diseases: a population-based case-control study. Sci Rep (2020) 10(1):18635. doi:10.1038/s41598-020-75815-x
33. Zhang, Z, Adappa, ND, Lautenbach, E, Chiu, AG, Doghramji, L, Howland, TJ, et al. The effect of diabetes mellitus on chronic rhinosinusitis and sinus surgery outcome. Int Forum Allergy Rhinol (2014) 4(4):315–20. doi:10.1002/alr.21269
34. di Coste, A, Costantino, F, Tarani, L, Savastano, V, Di Biasi, C, Schiavi, L, et al. Rhinocerebral zygomycosis with pansinusitis in a 14-year-old girl with type 1 diabetes: a case report and review of the literature. Ital J Pediatr (2013) 39:3977. doi:10.1186/1824-7288-39-77
35. Saraiva, M, and O'Garra, A. The regulation of il-10 production by immune cells. Nat Rev Immunol (2010) 10(3):170–81. doi:10.1038/nri2711
36. Ozenci, V, Kouwenhoven, M, Huang, YM, Xiao, B, Kivisäkk, P, Fredrikson, S, et al. Multiple sclerosis: levels of interleukin-10-secreting blood mononuclear cells are low in untreated patients but augmented during interferon-β-1b treatment. Scand J Immunol (1999) 49(5):554–61. doi:10.1046/j.1365-3083.1999.00546.x
37. Bettelli, E, Prabhu Das, M, Howard, ED, Weiner, HL, Sobel, RA, and Kuchroo, VK. Il-10 is critical in the regulation of autoimmune encephalomyelitis as demonstrated by studies of il-10- and il-4-deficient and transgenic mice. J Immunol (1998) 161(7):3299–306. doi:10.4049/jimmunol.161.7.3299
38. Psachoulia, K, Chamberlain, KA, Heo, D, Davis, SE, Paskus, JD, Nanescu, SE, et al. Il4i1 augments cns remyelination and axonal protection by modulating t cell driven inflammation. Brain (2016) 139(Pt 12):3121–36. doi:10.1093/brain/aww254
39. Cush, JJ, Splawski, JB, Thomas, R, Mcfarlin, JE, Schulze-Koops, H, Davis, LS, et al. Elevated interleukin-10 levels in patients with rheumatoid arthritis. Arthritis Rheum (1995) 38(1):96–104. doi:10.1002/art.1780380115
40. Bucht, A, Larsson, P, Weisbrot, L, Thorne, C, Pisa, P, Smedegård, G, et al. Expression of interferon-gamma (IFN-δ), IL-10, IL-12 and transforming growth factor-beta (TGF-β) mRNA in synovial fluid cells from patients in the early and late phases of rheumatoid arthritis (RA). Clin Exp Immunol (2007) 103(3):357–67. doi:10.1111/j.1365-2249.1996.tb08288.x
41. Bober, LA, Rojas-Triana, A, Jackson, JV, Leach, MW, Manfra, D, Narula, SK, et al. Regulatory effects of interleukin-4 and interleukin-10 on human neutrophil function ex vivo and on neutrophil influx in a rat model of arthritis. Arthritis Rheum (2000) 43(12):2660–7. doi:10.1002/1529-0131(200012)43:12<2660::AID-ANR5>3.0.CO;2-4
42. Tian, S, Yan, Y, Qi, X, Li, X, and Li, Z. Treatment of type ii collagen-induced rat rheumatoid arthritis model by interleukin 10 (il10)-mesenchymal stem cells (bmscs). Med Sci Monitor (2019) 25:252923–2934. doi:10.12659/MSM.911184
43. de la Vega, JR, Vilaplana, JC, Biro, A, Hammond, L, Bottazzo, GF, and Mirakian, R. Il-10 expression in thyroid glands: protective or harmful role against thyroid autoimmunity? Clin Exp Immunol (2001) 113(1):126–35. doi:10.1046/j.1365-2249.1998.00628.x
44. Mikoś, H, Mikoś, M, Obara-Moszyńska, M, and Niedziela, M. Rola układu immunologicznego oraz udział cytokin w patomechanizmie autoimmunologicznej choroby tarczycy (AITD). Endokrynologia Polska (2014) 65(2):150–5. doi:10.5603/EP.2014.0021
45. Fallahi, P, Ferrari, SM, Ragusa, F, Ruffilli, I, Elia, G, Paparo, SR, et al. Th1 chemokines in autoimmune endocrine disorders. J Clin Endocrinol Metab (2020) 105(4):1046–60. doi:10.1210/clinem/dgz289
46. Tsybikov, NN, Egorova, EV, Kuznik, BI, Fefelova, EV, and Magen, E. Anticytokine autoantibodies in chronic rhinosinusitis. Allergy Asthma Proc (2015) 36(6):473–80. doi:10.2500/aap.2015.36.3880
47. Li, L, Feng, J, Zhang, D, Yong, J, Wang, Y, Yao, J, et al. Differential expression of mir-4492 and il-10 is involved in chronic rhinosinusitis with nasal polyps. Exp Ther Med (2019) 18(5):3968–76. doi:10.3892/etm.2019.8022
48. Xuan, L, Zhang, N, Wang, X, Zhang, L, and Bachert, C. Il-10 family cytokines in chronic rhinosinusitis with nasal polyps: from experiments to the clinic. Front Immunol (2022) 13:13947983. doi:10.3389/fimmu.2022.947983
49. Han, X, Wu, D, Sun, Z, Sun, H, Lv, Q, Zhang, L, et al. Type 1/type 2 inflammatory cytokines correlate with olfactory function in patients with chronic rhinosinusitis. Am J Otolaryngol (2020) 41(5):102587. doi:10.1016/j.amjoto.2020.102587
50. Mantovani, A, Sica, A, Sozzani, S, Allavena, P, Vecchi, A, and Locati, M. The chemokine system in diverse forms of macrophage activation and polarization. Trends Immunol (2004) 25(12):677–86. doi:10.1016/j.it.2004.09.015
51. Mahdavinia, M, Keshavarzian, A, Tobin, MC, Landay, AL, and Schleimer, RP. A comprehensive review of the nasal microbiome in chronic rhinosinusitis (crs). Clin Exp Allergy (2016) 46(1):21–41. doi:10.1111/cea.12666
52. Ouyang, W, Rutz, S, Crellin, NK, Valdez, PA, and Hymowitz, SG. Regulation and functions of the il-10 family of cytokines in inflammation and disease. Annu Rev Immunol (2011) 29:2971–109. doi:10.1146/annurev-immunol-031210-101312
53. Alanärä, T, Karstila, K, Moilanen, T, Silvennoinen, O, and Isomäki, P. Expression of il-10 family cytokines in rheumatoid arthritis: elevated levels of il-19 in the joints. Scand J Rheumatol (2010) 39(2):118–26. doi:10.3109/03009740903170823
54. Hsu, Y, Hsieh, P, and Chang, M. Interleukin-19 blockade attenuates collagen-induced arthritis in rats. Rheumatology (Oxford, England) (2012) 51(3):434–42. doi:10.1093/rheumatology/ker127
55. Steiniche, T, Kragballe, K, Rømer, J, Hasselager, E, Nørby, PL, and Thorn Clausen, J. Epidermal overexpression of interleukin-19 and -20 mrna in psoriatic skin disappears after short-term treatment with cyclosporine a or calcipotriol. J Invest Dermatol (2003) 121(6):1306–11. doi:10.1111/j.1523-1747.2003.12626.x
56. Otkjaer, K, Kragballe, K, Funding, AT, Clausen, JT, Noerby, PL, Steiniche, T, et al. The dynamics of gene expression of interleukin-19 and interleukin-20 and their receptors in psoriasis. Br J Dermatol (2005) 153(5):911–8. doi:10.1111/j.1365-2133.2005.06800.x
57. Li, H, Lin, Y, Chen, P, Hsiao, C, Lee, JY, Chen, W, et al. Interleukin-19 upregulates keratinocyte growth factor and is associated with psoriasis. Br J Dermatol (2005) 153(3):591–5. doi:10.1111/j.1365-2133.2005.06665.x
58. Wang, H, Hsu, Y, and Chang, M. Il-20 bone diseases involvement and therapeutic target potential. J Biomed Sci (2018) 25(1):38. doi:10.1186/s12929-018-0439-z
59. Blumberg, H, Conklin, D, Xu, WF, Grossmann, A, Brender, T, Carollo, S, et al. Interleukin 20: discovery, receptor identification, and role in epidermal function. Cell (2001) 104(1):9–19. doi:10.1016/s0092-8674(01)00187-8
60. Stenderup, K, Rosada, C, Worsaae, A, Dagnaes-Hansen, F, Steiniche, T, Hasselager, E, et al. Interleukin-20 plays a critical role in maintenance and development of psoriasis in the human xenograft transplantation model. Br J Dermatol (2009) 160(2):284–96. doi:10.1111/j.1365-2133.2008.08890.x
62. Hao, J. Targeting interleukin-22 in psoriasis. Inflammation (2014) 37(1):94–9. doi:10.1007/s10753-013-9715-y
63. Zhong, Y, Zhang, X, and Chong, W. Interleukin-24 immunobiology and its roles in inflammatory diseases. Int J Mol Sci (2022) 23(2):627. doi:10.3390/ijms23020627
64. Ruffilli, I, Ferrari, SM, Colaci, M, Ferri, C, Fallahi, P, and Antonelli, A. Ip-10 in autoimmune thyroiditis. Horm Metab Res (2014) 46(9):597–602. doi:10.1055/s-0034-1382053
65. Kuan, WP, Tam, L, Wong, C, Ko, FWS, Li, T, Zhu, T, et al. Cxcl 9 and cxcl 10 as sensitive markers of disease activity in patients with rheumatoid arthritis. J Rheumatol (2010) 37(2):257–64. doi:10.3899/jrheum.090769
66. Godessart, N, and Kunkel, SL. Chemokines in autoimmune disease. Curr Opin Immunol (2001) 13(6):670–5. doi:10.1016/s0952-7915(01)00277-1
67. Spurrell, JCL, Wiehler, S, Zaheer, RS, Sanders, SP, and Proud, D. Human airway epithelial cells produce ip-10 (cxcl10) in vitro and in vivo upon rhinovirus infection. Am J Physiology-Lung Cell Mol Physiol (2005) 289(1):L85–95. doi:10.1152/ajplung.00397.2004
68. Corne, JM, and Holgate, ST. Mechanisms of virus induced exacerbations of asthma. Thorax (1997) 52(4):380–9. doi:10.1136/thx.52.4.380
69. Förster-Ruhrmann, U, Szczepek, AJ, Pierchalla, G, Fluhr, JW, Artuc, M, Zuberbier, T, et al. Chemokine expression-based endotype clustering of chronic rhinosinusitis. J Personalized Med (2022) 12(4):646. doi:10.3390/jpm12040646
70. Hassan, NJ, Simmonds, SJ, Clarkson, NG, Hanrahan, S, Puklavec, MJ, Bomb, M, et al. Cd6 regulates t-cell responses through activation-dependent recruitment of the positive regulator slp-76. Mol Cell Biol (2006) 26(17):6727–38. doi:10.1128/MCB.00688-06
71. Gonçalves, CM, Henriques, SN, Santos, RF, and Carmo, AM. Cd6, a rheostat-type signalosome that tunes t cell activation. Front Immunol (2018) 9:92994. doi:10.3389/fimmu.2018.02994
72. Pinto, M, and Carmo, AM. Cd6 as a therapeutic target in autoimmune diseases: successes and challenges. BioDrugs (2013) 27(3):191–202. doi:10.1007/s40259-013-0027-4
73. Havrdová, E, Belova, A, Goloborodko, A, Tisserant, A, Wright, A, Wallstroem, E, et al. Activity of secukinumab, an anti-il-17a antibody, on brain lesions in rrms: results from a randomized, proof-of-concept study. J Neurol (2016) 263(7):1287–95. doi:10.1007/s00415-016-8128-x
74. Mcinnes, IB, and Schett, G. Cytokines in the pathogenesis of rheumatoid arthritis. Nat Rev Immunol (2007) 7(6):429–42. doi:10.1038/nri2094
75. Nepom, GT, Ehlers, M, and Mandrup-Poulsen, T. Anti-cytokine therapies in t1d: concepts and strategies. Clin Immunol (Orlando, Fla.) (2013) 149(3):279–85. doi:10.1016/j.clim.2013.02.003
76. Setty, M, Discepolo, V, Abadie, V, Kamhawi, S, Mayassi, T, Kent, A, et al. Distinct and synergistic contributions of epithelial stress and adaptive immunity to functions of intraepithelial killer cells and active celiac disease. Gastroenterology (2015) 149(3):681–91.e10. doi:10.1053/j.gastro.2015.05.013
77. Cui, L, Chu, S, and Chen, N. The role of chemokines and chemokine receptors in multiple sclerosis. Int Immunopharmacology (2020) 83:83106314. doi:10.1016/j.intimp.2020.106314
78. Han, X, Li, L, Yang, J, King, G, Xiao, Z, and Quarles, LD. Counter-regulatory paracrine actions of fgf-23 and 1,25(oh)2 d in macrophages. FEBS Lett (2016) 590(1):53–67. doi:10.1002/1873-3468.12040
79. Emdin, CA, Khera, AV, and Kathiresan, S. Mendelian randomization. Jama (2017) 318(19):1925–6. doi:10.1001/jama.2017.17219
Keywords: autoimmune diseases, rhinosinusitis, inflammatory factors, mediating role, Mendelian randomization
Citation: Liang Y, Yin S, Chen X, Li C and Chen Q (2024) The causal relationship between autoimmune diseases and rhinosinusitis, and the mediating role of inflammatory proteins: a Mendelian randomization study. Exp. Biol. Med. 249:10196. doi: 10.3389/ebm.2024.10196
Received: 10 April 2024; Accepted: 12 June 2024;
Published: 22 July 2024.
Copyright © 2024 Liang, Yin, Chen, Li and Chen. This is an open-access article distributed under the terms of the Creative Commons Attribution License (CC BY). The use, distribution or reproduction in other forums is permitted, provided the original author(s) and the copyright owner(s) are credited and that the original publication in this journal is cited, in accordance with accepted academic practice. No use, distribution or reproduction is permitted which does not comply with these terms.
*Correspondence: Qiu Chen, Y2hlbnFpdTEwMDVAY2R1dGNtLmVkdS5jbg==